Like all forms of ionising radiation, X-rays produce electrons and ions when they pass through materials.
What are X-rays?
An X-ray is a packet of electromagnetic energy (photon) that originate from the electron cloud of an atom. This is generally caused by energy changes in an electron, which moves from a higher energy level to a lower one, causing the excess energy to be released. X-rays are similar to gamma rays however the main difference is the way they are produced, X-rays are produced by electrons external to the nucleus. Traditionally X-rays had longer-wavelengths and lower energy than gamma rays but this is obsolete with modern X-ray production methods.
What are the properties of X-rays?
X-rays are a form of electromagnetic radiation similar to radio waves, microwaves, visible light and gamma rays. X-ray photons are highly energetic and have enough energy to break up molecules and hence damage living cells. When X-rays hit a material some are absorbed and others pass through. Generally, the higher the energy the more X-rays will pass through (Table 1). It is this penetrating power that allows us to take internal images of the human body or objects. X-rays cannot be steered by electric and magnetic fields like alphas, betas or other charged particles.
Table 1
Energy carried by each photon | Frequency of electromagnetic wave (Hz) | Wavelength (pm, 1pm = 10 -12m) | Thickness of material to halve number of photons (half value thickness) (mm) | ||||
---|---|---|---|---|---|---|---|
in electron-volts (eV) | in joules (J) | Concrete | Lead | Human tissue | Aluminium | ||
1keV | 1.602 X 10 -16 | 2.418 X 10 17 | 1240 | 0.0009 | 0.00012 | 0.0018 | 0.0022 |
10keV | 1.602 X 10 -15 | 2.418 X 10 18 | 124 | 0.147 | 0.047 | 1.22 | 0.098 |
100keV | 1.602 X 10 -14 | 2.418 X 10 19 | 12.4 | 17.3 | 0.110 | 38.6 | 15.1 |
1MeV | 1.602 X 10 -13 | 2.418 X 10 20 | 1.24 | 46.4 | 8.60 | 93.3 | 41.8 |
10MeV | 1.602 X 10 -12 | 2.418 X 10 21 | 0.124 | 132 | 12.3 | 298 | 111 |
What are the health effects of exposure to X-rays?
X-rays are highly penetrating and interact with matter through ionisation via three processes, photoelectric effect, Compton scattering or pair production. Due to their high penetration power the impact of X-rays can occur throughout a body, they are however less ionising than alpha particles. X-rays are considered an external hazard with regards to radiation protection.
Similar to all exposure to ionising radiation high exposures can cause direct acute effects through immediate damage to cells. Low levels of exposure carry a stochastic health risk where the probability of cancer induction increases with increased exposure.
What is the difference between gamma rays and X-rays?
The key difference between gamma rays and X-rays is how they are produced. Gamma rays originate from the settling process of an excited nucleus of a radionuclide after it undergoes radioactive decay whereas X-rays are produced when electrons strike a target or when electrons rearrange within an atom.
What are some common sources of X-rays?
X-rays are commonly produced in X-ray tubes by accelerating electrons through a potential difference (a voltage drop) and directing them onto a target material (i.e. tungsten).
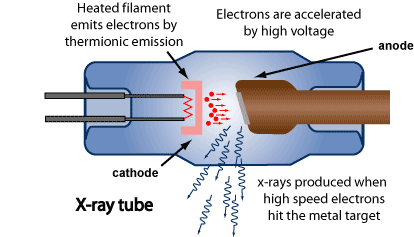
The incoming electrons release X-rays as they slowdown in the target (braking radiation or bremsstrahlung). The X-ray photons produced in this manner range in energy from near zero up to the energy of the electrons. An incoming electron may also collide with an atom in the target, kicking out an electron and leaving a vacancy in one of the atom’s electron shells. Another electron may fill the vacancy and in so doing release an X-ray photon of a specific energy (a characteristic X-ray). The X-ray spectrum shown in the picture is a plot of the number of photons against the photon energy.
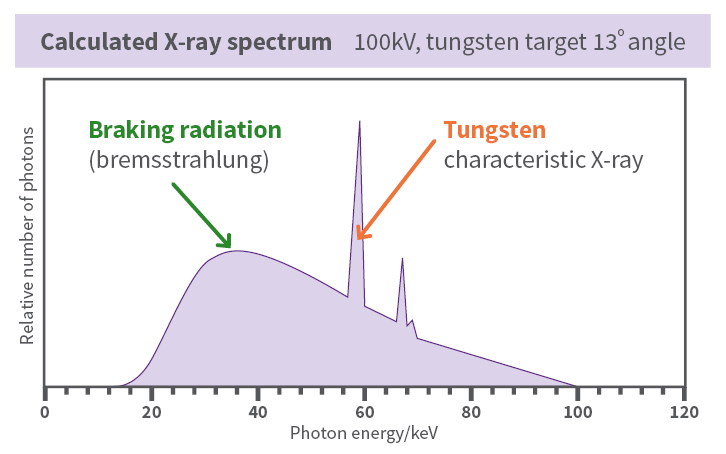
A computed tomography (CT) scanner is a particular type of X-ray machine in which the X-ray tube produces a beam in the shape of a fan and moves around the patient in a circle. The X-rays are detected electronically and a computer uses the information to reconstruct an image of the region of the body exposed.
X-rays can also be produced by a synchrotron. A synchrotron is a device that accelerates electrons in an evacuated ring (often several tens of metres in diameter), steering them with magnets. Manipulating the electron beam in a controlled way with the magnets can produce intense beams of X-rays. Synchrotron facilities are used for research purposes.
What are some uses of X-rays?
X-rays have a large range of uses for medical, industrial and research purposes. Diagnostic medical X-rays are the most likely way you will encounter X-rays. Data from the Health Insurance Commission shows that each year there are over 12 million Medicare claims for exams on X-ray machines and also over 2 million claims for computed tomography (CT) exams. Radiotherapy is another example of the medical use of X-rays to treat cancer.
On average, each Australian receives an effective dose of about 1.7 mSv per year from medical procedures, including about 1.1 mSv from CT scans. This is similar to the dose everyone receives from background radiation that is and always has been in our environment.
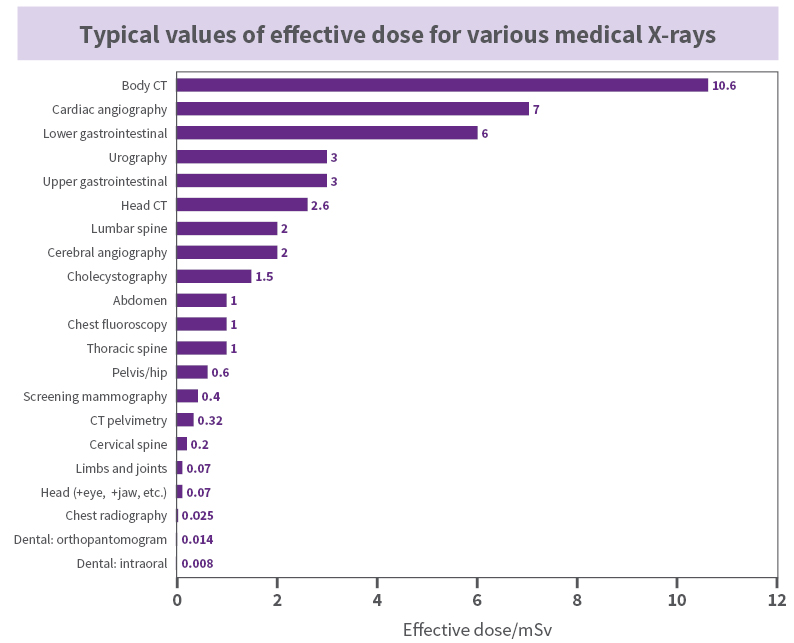
Industrial and research uses of X-rays include X-ray crystallography and fluoroscopy which are commonly used for the quality control of materials (i.e. metal quality) and investigating the properties of materials. Industrial radiography can use X-ray or gamma sources for analysis to look for cracks in buildings, structures or pressure vessels.
X-rays are also used in security processes for baggage/container screening at airports and ports.
The accelerating voltage and the target material used to produce X-rays vary depending on the particular application (Table 2).
Table 2
Use | Accelerating potential | Target | Source type | Average photon energy | |
---|---|---|---|---|---|
X-ray crystallography | 40 kV 60 kV | Copper Molybdenum | Tube | 8 keV - 17 keV | |
Diagnostic X-rays | Mammography | 26 - 30 kV | Rhodium Molybdenum | Tube | 20 keV |
Dental | 60 kV | Tungsten | Tube | 30 keV | |
General | 50 - 140 kV | Tungsten | Tube | 40 keV | |
CT | 80 - 140 kV | Tungsten | Tube | 60 keV | |
Baggage screening | Carry-on/checked bags | 80 - 160kV | Tungsten | Tube | 80keV |
Container screening | 450kV - 20MV | Tungsten | Tube/Linear accelerator | 150keV - 9MeV | |
Structural analysis | 150 - 450 kV | Tungsten | Tube | 100keV | |
X-ray therapy | 10 - 25 MV | Tungsten/High Z material | Linear accelerator | 3 - 10 MeV |
How can I minimise my exposure to X-rays?
As with all types of radiation, the protection principles are time, distance and shielding.
Those using X-rays at work (e.g. medical staff, laboratory workers) should minimise the time they spend near the source and move further away if they don’t need to be close. Shielding structures, both fixed and mobile, can be used to reduce exposure. As lead is a very good attenuator of X-rays (see Table 1), a garment impregnated with a small amount of lead can be used to shield the body. Other heavy elements can also be used and in some cases these permit adequate protection with a much lighter garment, which can reduce the strain on the wearer.
For patients, diagnostic X-ray imaging should only be performed to provide information that helps medical staff treat a patient’s condition appropriately. This information is much more important to a person’s health than the very small potential chance of developing cancer from the exposure. Modern X-ray equipment has many control features that if used properly can limit the area irradiated and the dose delivered to the minimum necessary for obtaining the diagnostic information. Shielding for patients is typically not necessary if these control features are used appropriately and may even interfere with the proper functioning of these controls. In some cases, an alternative type of imaging (ultrasound or magnetic resonance imaging) may be able to provide the information sought and therefore may be used instead of X-rays.